Combining topology and cosmology to detect Dark Matter
The Alexander von Humboldt Sofia Kovalevskaja group at the Max Planck Institute of Microstructure Physics, led by Dr. Mazhar Ali, published a new manuscript in Physical Review Letters detailing a new method for detecting Dark Matter and a route to constructing a “table-top” Dark Matter detector.
One of the great unsolved mysterious in all of science is the question of what Dark Matter is. While we luckily live in a pocket of visible matter, we know that the vast majority of matter in the universe is in fact “dark”. Non-luminous matter comprises 84% of the mass of the known universe as measured by the angular structure of the Cosmic Microwave Background [1]. There are many theories as to what types of particles make up Dark Matter, ranging from “dark electrons” to Gravitrinos to WIMPs (Weakly Interacting Massive Particles).
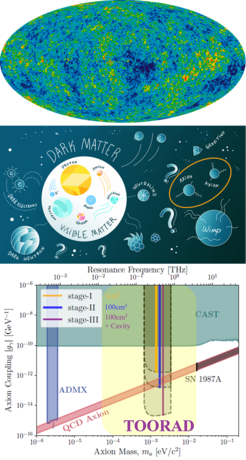
In order to test these theories, many experimental efforts are underway with the majority focused on WIMPS. These have been large-scale experiments like LUX, XENON, and PandaX-II (in the US, Italy, and China respectively) [2] which looked for WIMPs to collide with xenon nuclei, causing nuclear recoils which would be detectable using photomultiplier tubes and multi-array detectors in large, cryogenic cavities capable of holding hundreds of kilograms of liquid Xe. To date, no one has detected dark matter despite years of effort; however, the efforts were not in vain. Knowing the lower-limit of the detection capabilities of each experiment sets hard constraints on what mass/coupling regime dark matter must be hiding in and helps inform the next experimental effort.
Today, many scientists are shifting focus towards the Dark Axion, another suggestion for comprising dark matter. Contrary to the WIMP, the Dark Axion is believed to be an ultralight particle and many new experiments have turned on in recent years including ADMX, CAST, MADMAX, and others [1,3,4]. The Axion was first predicted by Nobel laureate Frank Wilzcek (2004 Physics) in order to solve the strong CP symmetry violation problem in particle physics, which would have led to the conclusion that Neutrons should have an electrical dipole [5, 6]. The Axion was proposed in order to remedy this problem by essentially “absorbing” fluctuations in θ, the term responsible for the violations, which is also an indicator of the topological state of electrons in a crystalline material.
In this paper, Marsh et al, seize on this fact and realize that crystals or films of topological insulators, under special circumstances, can be used to detect the Dark Axion. Magnetic topological insulators (a.k.a. axionic insulators) can host axion quasiparticles whose masses can be tuned using an applied magnetic field. When the mass of the analog axion is the same as the Dark Axion, they will resonantly couple and emit light towards a photon detector.
The authors propose to build TOORAD, a “table-top” dark matter experiment using this concept. Such an experiment can probe an area of the mass/coupling space which no other experiment or proposal has ever been able to scan, including the especially difficult “QCD Axion” area which was theorized long ago. Their research was published recently in Physical Review Letters.
Read the paper at: https://doi.org/10.1103/PhysRevLett.123.121601
Proposal to Detect Dark Matter using Axionic Topological Antiferromagnets
Abstract: Antiferromagnetically doped topological insulators (ATI) are among the candidates to host dynamicalaxion fields and axion polaritons, weakly interacting quasiparticles that are analogous to the dark axion, along sought after candidate dark matter particle. Here we demonstrate that using the axion quasiparticle antiferromagnetic resonance in ATIs in conjunction with low-noise methods of detecting THz photons presents a viable route to detect axion dark matter with a mass of 0.7 to 3.5 meV, a range currently inaccessible to other dark matter detection experiments and proposals. The benefits of this method at high frequency are the tunability of the resonance with applied magnetic field, and the use of ATI samples with volumes much larger than 1mm3.
References:
1. N. A. Bahcall, Proceedings of the National Academy of Sciences, 112, 40, 12243 (2015).
2. Liu et al, Nature Physics, 13, 212-216 (2017).
3. CAST collaboration, Nature Physics, 13, 584-590 (2017).
4. MADMAX working group et al, Physical Review Letters, 118, 091801 (2017).
5. L. D. Duffy et al, New Journal of Physics, 11 (2009).
6. R. D. Peccei, et al, Physical Review Letters, 38, 1440 (1977)
7. http://www.symmetrymagazine.org/