Energy Materials and Devices
Dr. Minghao Yu
Research Area I. Next-generation Energy Materials
Novel Carbon Nanostructures

Novel carbon materials with tailor-made structures (e.g., topological defect, porosity, dopant, morphology) are of significant interest for a variety of energy-related applications. We are seeking for the development of novel structurally well-defined carbon nanostructures. The fundamental understanding of carbon growth mechanism, and new physical/chemical/electrochemical properties will be explored in the context of our research.
2D Redox-Active Carbon-Rich Frameworks

2D carbon-rich frameworks (including carbon-conjugated metal-organic frameworks (MOFs) and covalent organic frameworks (COFs)) have emerged as a group of intelligent multi-functional materials, owing to the designable topologies at the molecular level, regular porosities, and large specific surface areas. These materials offer great opportunities to design and synthesize new energy storage electrodes by organizing redox-active monomers into the periodic framework structures. We are highly interested in the design and synthesis of new framework molecules, as well as the investigation of these molecules for the next-generation energy storage technologies.
2D Materials & Van der Waals Heterostructures

The large family of atomically thin 2D materials (e.g., Graphene, Phosphorene, MXenes, TMDs, 2D organic materials) collectively covers a very broad range of chemical and physical properties. Van der Waals interaction between distinct 2D materials shows considerable compatibility, allowing the flexible assembly of 2D heterostructures that can synergize each building block's desirable properties. We are particularly interested in developing controllable synthetic routes, surface/defect chemistry control, unique/exceptional physicochemical properties, and energy applications of novel 2D materials and their heterostructures.
Research Area II. Energy Storage
Supercapacitors and Hybrid-Ion Capacitors

Supercapacitors, which are power-featured energy storage devices, deliver a power density that is one order of magnitude larger than that of lithium-ion batteries. Hybrid-ion capacitors represent one type of emerging energy storage devices, which are made up of a battery-type electrode and a capacitor-type electrode. To achieve high-performance supercapacitor/hybrid-ion capacitor devices, we aim at fabricating thin-film electrodes with large accessible electrochemically activated surfaces, high electrical conductivity, and an elaborate device structure with efficient ion diffusion pathways.
Dual-Ion Batteries
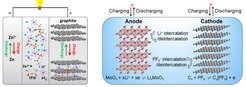
Anion-storage chemistry provides the possibility to enable battery cathodes with high potential windows (e.g., anion-intercalation graphite with an average discharge potential of > 4.5 V vs. Li+/Li). It opens up new application opportunities for constructing plenty of dual-ion batteries (DIBs), which significantly contrast with the conventional ‘rocking-chair’ Li-ion batteries. We are interested in the development of new anion-storage materials, rational electrolyte preparation, and novel dual-ion battery configuration design.
Multi-Valent Metal-Ion Batteries

Multivalent metal-ion chemistry offers feasible pathways to develop next-generation energy storage technologies with higher energy density, better safety, and lower cost, as the corresponding metals (e.g., Zn, Mg, Al) can be directly used as ideal multielectron-redox anodes. The main challenge of multivalent metal-ion batteries lies in the large difficulty of multivalent metal ions to be stored in convention battery materials, due to the large ionic size and strong repulsive intercalation with host materials. Our research efforts focus on developing high-performance cathode materials for accommodating multivalent metal ions, innovative approaches to alleviate the sluggish ion-storage kinetics, and construction of high-performance battery devices
Functional Devices & Smart Integration

The development of many cutting-edge technologies (e.g., wearable/implantable healthcare devices, environmental monitoring, internet of things) triggers the development of modern electronics towards miniaturized, portable, multi-functional, and highly integrated device systems. High demand for power sources with the same features is imposed, as traditional energy storage devices (e.g., supercapacitors and batteries) may not be of suitable choices. In this regard, we are highly interested in developing miniaturized energy storage devices (micro-supercapacitors and micro-batteries), introducing multi-functionalities into energy storage devices (e.g., mechanically flexibility/stretchability, sensing functions, stimulus-responsive functions), and constructing highly integrated self-powered energy systems.
Research Area III. Energy Conversion
Electrocatalytic Oxygen Reactions (Metal-Air Batteries, Fuel Cells)
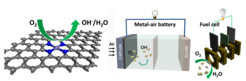
The increasing energy crisis and environmental pollution require innovative solutions for the use of renewable energies. Electrocatalytic oxygen reactions (OER, ORR) are the vital process for next-generation electrochemical energy storage and conversion technologies, e.g., metal-air batteries and fuel cells. Due to their earth-abundance and unique physicochemical properties, carbon-rich nanomaterials can serve as novel materials and electrodes for catalyzing the sluggish oxygen reaction kinetics. In order to achieve high catalytic performance in energy storage and conversion systems, we primarily focus on synthesizing novel carbon-based catalysts with improved intrinsic activity and favorable porous structures for mass transport.
Photoelectrochemical Water Splitting

Due to the high energy density and clean combustion product, hydrogen (H2) has been universally proposed as a promising energy carrier for future energy conversion and storage devices. Conjugated polymers, featuring tunable band gaps/positions and tailored active centers at the molecular level, are attractive photoelectrode materials for energy conversion. Developing conjugated polymers with small bandgaps, high separation capability of photoinduced holes and electrons, and highly active catalytic centers, provides a promising pathway for photoelectrochemical hydrogen/oxygen evolution reactions.
Nanofluidic Osmotic Power Generation

The osmotic energy between river water and seawater, also known as salinity gradient energy or blue energy, has been identified as promising clean, renewable, and non-intermittent sources of energy. Currently, the industrial utilization of such worldwide energy is mainly limited by the low performance of the commercial ion-exchange membrane. The unique electrolyte transport phenomena in the 2D nanofluidic channel system open a new avenue to harvest osmotic energy. We primarily focus on the design and construction of novel nanofluidic membranes based on synthetic 2D materials, investigation of the ion transport behaviour, and exploration of their application in osmotic power generation.