Workshop on "Transformational Materials II: Computational Material Design"
Workshop
- Start: Oct 7, 2021 02:15 PM (Local Time Germany)
- End: Oct 8, 2021 06:15 PM
- Speakers: Prof. Frank Ortmann, Prof. Kristian Sommer Thygesen, Prof. André Schleife, Prof. Evan Reed, Prof. Michele Ceriotti, Prof. Aleksandra Vojvodic, Prof. Kristin Persson
- Location: Max-Planck-Institut für Mikrostrukturphysik, Weinberg 2, 06120 Halle (Saale)
- Room: Online / Please click here to register in advance
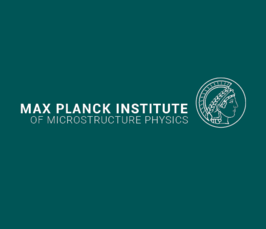
Program
DAY 1: October 7th, 2021
02:15 p.m. - Introduction
02:30 p.m. - From Charge and Spin Transport to Excitons in Solar Cells: Insights from Linear-Scaling Approaches - Prof. Frank Ortmann, Technical University of Munich, Germany
03:30 p.m. - High-Throughput Modeling and Discovery of Atomically Thin Crystals - Prof. Kristian Sommer Thygesen, Technical University of Denmark, Lyngby, Denmark
04:30 p.m. - Coffee Break
05:00 p.m. - Triggering Ion Dynamics in Materials By Laser and Particle Radiation - Prof. André Schleife, University of Illinois Urbana-Champaign, U.S.A.
06:00 p.m. - Synthesizable yet Unsynthesized Low-Dimensional Materials Revealed by Data Science - Prof. Evan Reed, Stanford University, Stanford, U.S.A.
DAY 2: October 8th, 2021
02:15 p.m. - Introduction
02:30 p.m. - Physics-Inspired Machine Learning for Molecular and Materials Modeling - Prof. Michele Ceriotti, EPFL, Lausanne, Switzerland
03:30 p.m. - Modeling Chemistry of Compound Materials for Catalysis and Energy - Prof. Aleksandra Vojvodic, University of Pennsylvania, Philadelphia, U.S.A.
04:30 p.m. - Coffee Break
05:00 p.m. - The Era of Data-driven Materials Innovation and Design - Prof. Kristin Persson, University of California at Berkeley, U.S.A.
06:00 p.m. - Wrap up
From charge and spin transport to excitons in solar cells: Insights from linear−scaling approaches
The number of interesting electronic materials is constantly growing and completely new classes are emerging that were unknown a few years ago, including topological insulators, Weyl semimetals, 2D materials and novel organic semiconductors. For such emerging materials, electronic-structure codes describe reliably their electronic properties that can be directly compared with suitable measurements.
However, understanding electron transfer and charge transport in functional materials is more challenging. This is because vibrations and disorder affect the propagation of electrons in a complex manner. In addition, simulations of large systems are often required, since the transport properties depend on the size of the system or because of large unit cells as for instance in polycrystalline or Moiré systems. Unfortunately, current tools suffer from a lack of accuracy or scale badly with the system size.
In this presentation, I will introduce linear-scaling approaches that can close this gap. I will demonstrate, for selected cases, how large-scale simulations in combination with ab initio electronic-structure methods can lead to an in-depth understanding of charge-transport and spin-transport signatures that would otherwise remain unexplained and describe other material properties reliably.
Prof. Frank Ortmann
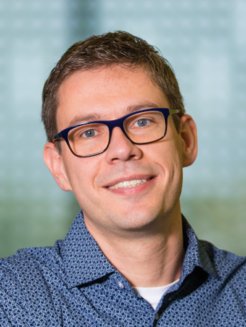
Technical University of Munich, Germany
Frank Ortmann received his doctorate with “summa cum laude” from the Friedrich Schiller University Jena (Germany) in 2009 and joined CEA Grenoble (France) as postdoctoral fellow in the same year where he was awarded a Marie-Curie Fellowship. In 2011 he moved to the Catalan Institute of Nanoscience and Nanotechnology, Barcelona (Spain). In 2013 he joined TU Dresden (Germany), where he headed an Emmy Noether young investigator group. Since 2020, he is assistant professor for “Theoretical Methods in Spectroscopy” at TU Munich.
High-Throughput Modeling and Discovery of Atomically Thin Crystals
Atomically thin two-dimensional (2D) materials represent a rapidly moving frontier of condensed matter physics. The reduced phase space and low dielectric screening equip these extremely thin materials with unique physical properties, which can be further tuned by external probes or by stacking the 2D materials into van der Waals heterostructures. In this talk, I will discuss how automated computational workflows can be used to characterize prospect 2D materials from first principles and how they can help to identify novel compounds with interesting properties such as magnetic order, non-trivial band topologies, and strong (non)linear optical response. Our high-throughput calculations are performed using the Atomic Simulation Recipes (ASR), which is a recently developed Python framework for constructing and working with computational workflows. In the last part of the talk, I will zoom in on the fascinating physics of 2D excitons. I will describe new types of excitonic states in 2D semiconductor heterostructures and illustrate how dielectric engineering provides an elegant means to modify – both quantitatively and qualitatively – the electronic excitations in a 2D materials without compromising the structural integrity of the material.
Prof. Kristian Sommer Thygesen
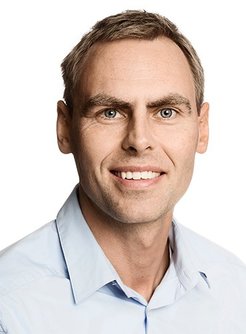
Technical University of Denmark, Lyngby, Denmark
Prof. Thygesen develops and applies first-principles methods based on density functional theory and many-body perturbation theory to describe the electronic structure of materials with a particular focus on nanostructured and low-dimensional materials. He is also interested in data-driven approaches to materials design and the development of automatized high-throughput workflow software. Prof. Thygesen is heading the section for Computational Atomic-scale Materials Design (CAMD) at the Technical University of Denmark, where he co-develops the GPAW electronic structure code, the Atomic Simulation Environment (ASE), and the Computational Materials Repository (CMR).
Triggering Ion Dynamics in Materials By Laser and Particle Radiation
Materials manipulation via ion or laser beams can achieve precisely tuned atomic geometries that are necessary, e.g. to engineer interactions between defects in quantum materials and for fabricating novel electronic devices with nanoscale dimensions. In addition, such beams are also used to characterize and probe materials properties by means of electronic and optical excitations. I will discuss recent quantum- mechanical first-principles predictions for electron dynamics and the subsequent ionic motion that follows after an excitation of the electronic system. Using real-time time-dependent density functional theory we simulated the underlying ultrafast time scales of electron dynamics in semiconductors and metals. Examples include long-lived electronic excitations in proton, electron, and laser irradiated bulk semiconductors that facilitate diffusion of point defects, such as oxygen vacancies in MgO. We compare such bulk simulations to aluminum surfaces under irradiation, for which we quantify electron emission, charge capture, and pre-equilibrium effects that are unique to thin films or two-dimensional materials. Limitations and possible extensions of the theoretical description will be included in the discussion.
Prof. André Schleife
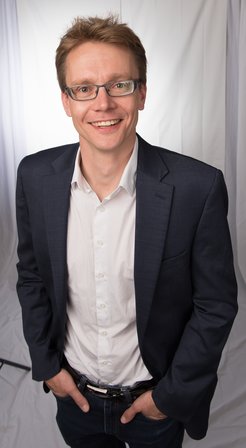
University of Illinois, Urbana-Champaign, U.S.A.
André Schleife is a Blue Waters Associate Professor in Materials Science and Engineering. He obtained his Diploma and Ph.D. at Friedrich-Schiller-University in Jena, Germany for theoretical and computational work on transparent conducting oxides. He then worked as a Postdoctoral Researcher at Lawrence Livermore National Laboratory on non-adiabatic electron-ion dynamics. He received the NSF CAREER award, the ONR YIP award, and was an ACS PRF doctoral new investigator. André is editor for a journal and actively organizes national and international schools, workshops, and tutorials
Synthesizable yet Unsynthesized Low-Dimensional Materials Revealed by Data Science
It is a grand challenge to predict the existence of stable and useful materials that have yet to be created in the lab. Some of the complicating factors include the role of metastability and kinetic effects, and environmental stability with respect to air and potential contaminants. Here we augment experimental synthetic data1 with physics-based machine learning to discover the chemical composition of more than 1000 materials that are likely to exhibit layered and two-dimensional phases but have yet to be synthesized.2 This includes two materials our calculations indicate can exist in distinct structures with different band gaps, expanding the short list of two-dimensional phase change materials. We find our model performs five times better than practitioners in the field at identifying layered materials and is comparable or better than professional solid-state chemists. Finally, we find that semi-supervised learning can offer benefits for materials design where labels for some of the materials are unknown.
Prof. Evan J. Reed
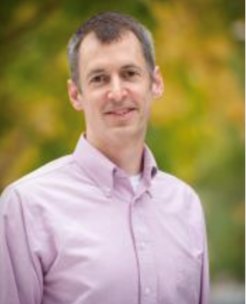
Stanford University, U.S.A.
Evan Reed is a faculty member in Materials Science and Engineering at Stanford University. He received a B.S. in applied physics from Caltech (1998) and PhD. in physics from MIT (2003). In 2004, he was an E. O. Lawrence Fellow and staff scientist at Lawrence Livermore National Laboratory before moving to Stanford in 2010. Evan Reed’s recent work focuses on atomic scale theory and modeling of 2D and other electronic materials, statistical learning for chemical and energy storage applications, structural phase changes for PCM, and high pressure shock wave compression.
Physics-Inspired Machine Learning for Molecular and Materials Modeling
Machine learning is making its way into all fields of science, and it is clear that - beyond the hype - it provides useful tools that are already changing the way both experiments and modeling are performed.
In particular, statistical regression techniques have become very fashionable as a tool to predict the properties of systems at the atomic scale, sidestepping much of the computational cost of accurate quantum chemical calculations, and making it possible to perform simulations that require thorough statistical sampling without compromising on the accuracy of the electronic structure model.
In this talk I will argue how data-driven modelling can be rooted in a mathematically rigorous and physically-motivated framework, and discuss the benefits of such a principled approach.
I will present several examples demonstrating how the combination of machine-learning and atomistic simulations can offer useful insights on the behavior of complex systems, and discuss the challenges towards an integrated modeling framework in which physics- and data-driven steps can be combined to improve the accuracy, the computational efficiency and the transferability of predictions.
Prof. Michele Ceriotti

EPFL, Lausanne, Switzerland
Michele Ceriotti received his Ph.D. in Physics from ETH Zürich. He spent three years in Oxford as a Junior Research Fellow at Merton College.
Since 2013 he leads the laboratory for Computational Science and Modeling, in the institute of Materials at EPFL.
His research interests focus on methods for molecular dynamics and quantum simulations, the development of machine-learning techniques for the study of complex systems at the atomistic level, and their application to problems in chemistry and materials science.
He has been awarded the IBM Research Forschungspreis in 2010, the Volker Heine Young Investigator Award in 2013, an ERC Starting Grant in 2016, the IUPAP C10 Young Scientist Prize in 2018, and an ERC Consolidator grant in 2021.
Modeling Chemistry of Compound Materials for Catalysis and Energy
The race to solve the sustainable energy puzzle is on. First-principles based computational methods, which balance accuracy and computational cost, are at the forefront for solving this puzzle. These computational approaches allow one to describe and understand chemistries of already known materials, and, importantly, they can be used to predict new materials through a careful analysis of the surface chemistry and electronic structure at the atomic level. In this talk, I will demonstrate how we have been able to computationally predict several new complex catalyst materials including metal oxides, carbides and nitrides which have been experimentally synthesized, characterized and tested. I then share recent insights on the stability and activity of computationally predicted nanostructured catalyst-systems. I conclude with new efforts to understand the dynamic behavior of these complex compound materials.
Prof. Aleksandra Vojvodic

Dr. Aleksandra Vojvodic is the Rosenbluth Associate Professor at the Department of Chemical and Biomolecular Engineering and the Director of Penn Institute of Computational Science at the University of Pennsylvania. Her research focuses on theoretical and computational-driven materials design, in particular on studies of surfaces and interfaces of complex materials for chemical transformations and energy conversion and storage.
In 2020, she was recognized as one of the “Women Scientists at the Forefront of Energy Research”. She is the recipient of the Mellichamp Distinguished Lecture, Young Innovator Award in NanoEnergy, the European Federation of Catalysis Societies (EFCATS) Young Researcher Award and of the MIT Technology Review 35 Award which recognized her work and innovative approaches and identified her as “[a] computation whiz that speeds up the search for catalysts that will make green chemistry possible”. She has been selected as a CIFAR Bio-Inspired Solar Energy program fellow and Scialog fellow in Advanced Energy Storage. She received her Ph.D. in Physics from the Department of Applied Physics at Chalmers University of Technology and her Master of Science in Physics from Lund University in Sweden.
The Era of Data-driven Materials Innovation and Design
Fueled by our abilities to compute materials properties and characteristics orders of magnitude faster than they can be measured and recent advancements in harnessing literature data, we are entering the era of the fourth paradigm of science: data-driven materials design. The Materials Project (www.materialsproject.org) uses supercomputing together with state-of-the-art quantum mechanical theory to compute the properties of all known inorganic materials and beyond, design novel materials and offer the data for free to the community together with online analysis and design algorithms. The current release contains data derived from quantum mechanical calculations for over 100,000 materials and millions of associated properties. The resource supports a growing community of data-rich materials research, currently comprising over 200,000 registered users and between 2-5 million data records served each day through the API. The software infrastructure enables thousands of calculations per week – enabling screening and predictions - for both novel solid as well as molecular species with target properties. However, truly accelerating materials innovation also requires rapid synthesis, testing and feedback. The ability to devise data-driven methodologies to guide synthesis efforts is needed as well as rapid interrogation and recording of results – including ‘non-successful’ ones. In this talk, I will highlight some of our ongoing work, including efficient harnessing of community data together with our own computational data enabling iteration between ideas, new materials development, synthesis and characterization as enabled by new algorithmic tools and data-driven approaches.
Prof. Kristin Persson

Kristin Persson is the Director of the Molecular Foundry, a user facility at Lawrence Berkeley National Lab (LBNL), a Professor at the University of California, Berkeley and a Faculty Senior Scientist at LBNL. She is the Director and co-founder of the Materials Project (www.materialsproject.org); one of the most visible of the Materials Genome Initiative (MGI) funded programs attracting >200,000 users worldwide with thousands of unique users accessing the site every day. She is a leader in the MGI community, and applies modeling and data-driven methodologies to the innovation of materials for energy storage and production. She serves in several scientific leadership roles, including in the Department of Energy’s Joint Center for Energy Storage Research Center (JCESR) and the Battery Materials Research (BMR) Program. She serves as an Associate Editor for Chemistry of Materials, on the advisory board of NanoHub and FAIRmat and she is the appointed MGI ambassador for The Metal, Minerals, and Materials Society (TMS). She has received the 2018 DOE Secretary of Energy’s Achievement Award, the 2017 TMS Faculty Early Career Award, the 2020 Falling Walls Science and Innovation Management Award, the LBNL Director’s award for Exceptional Scientific Achievement (2013), she is a 2018 Kavli Fellow and, as of 2020, is a top 1% cited researcher (Web of Science). She holds several patents in the clean energy space and has co-authored more than 200 peer-reviewed publications (h-index 76). Persson obtained her Ph.D. in Theoretical Physics at the Royal Institute of Technology in Stockholm, Sweden in 2001.